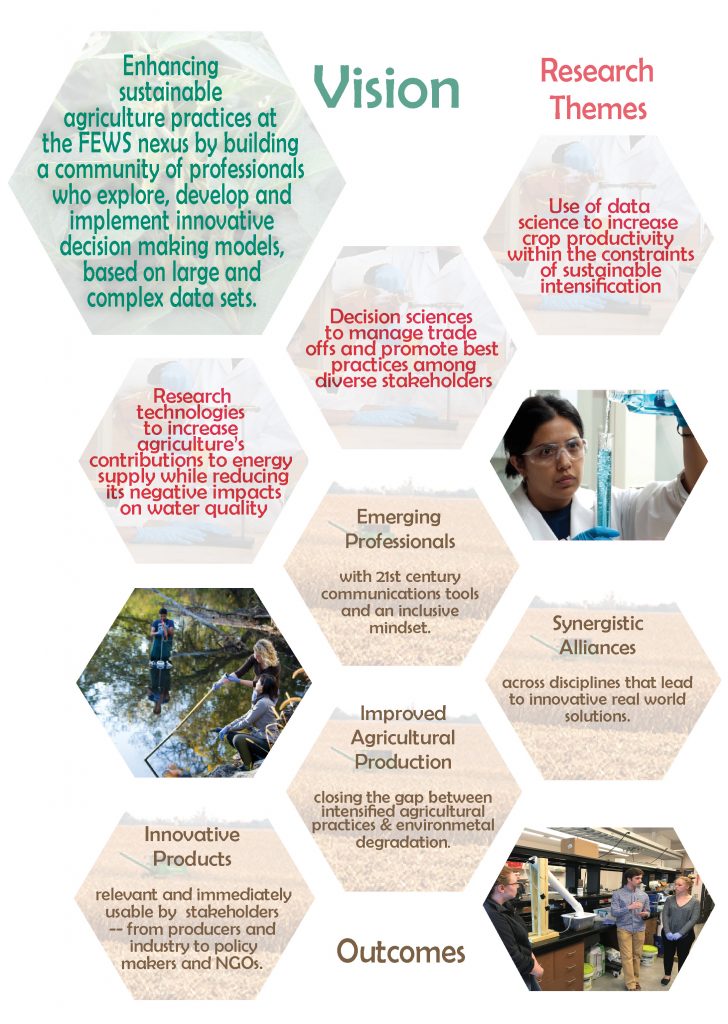
Major Research Efforts
Midwest agriculture contributes to both food and energy supply, the latter via the production of biofuels, mostly ethanol from corn. Increasingly, cellulosic biofuels are also produced from crop residues and dedicated energy crops that can be profitably grown on land not well-suited for food production. Water is used for biofuel production but, more importantly for much of the Midwest, runoff of nitrogen (N) and phosphorus (P) from fertilizers, including manure, affect water quality. Extensive use of N significantly increases leaching of nitrate (NO3) to the groundwater. Nitrate-nitrogen transported to the Gulf of Mexico contributes to the Dead Zone, a region of hypoxic conditions inhospitable to fish. Phosphorus drives harmful algae blooms in local waterbodies and also contributes to algal blooms in the Gulf, but its pathways are less understood.
Excess nitrate concentrations pose a threat to human health (i.e., “Blue Baby Syndrome” and certain types of cancer). Antimicrobial resistant bacteria and resistance genes pose another health threat caused by the application of manure from animal production facilities and spread by runoff.
Extensive rainfed croplands that predominantly produce annual grain crops for animal feed and ethanol are common to the Midwest and exemplified in Iowa. According to the USDA [13], Iowa ranks first in the nation in harvested acres of principal crops and corn grain production, and second in total value of agricultural products sold – both crops and livestock/poultry. It leads the nation in numbers of laying hens and pullets as well as hogs and pigs and falls in the top ten for turkeys, cattle, and calves. Iowa is the epicenter of corn-based ethanol production for biofuel, representing almost a third of the US’s ethanol capacity. Ethanol production in the state increased by 760% between 2000 and 2014. This robust agricultural and energy economy is a critical component of the state’s economy, the nation’s food supply, and the nation’s renewable fuel supply, but puts significant pressure on water supplies locally and far downstream. The focus on starch-derived ethanol and loss of soil has caused a decline in corn grain quality.
Average annual export of nitrate in surface/baseflow water from Iowa contributes 25% of the nitrate load to the Gulf, despite the state occupying less than 5% of the Mississippi River watershed [14]. Iowa’ s disproportionate contribution stems from productive farmland and widespread subsurface drainage, which removes excess water from the root zone but also provides a pathway for dissolved nitrate. These are also critical concerns locally. In 2015, the Des Moines Water Works (DMWW), servicing more than 500,000 customers in central Iowa and operating the largest nitrate removal facility in the world, filed a lawsuit against the county supervisors who oversee the drainage districts, alleging failures in regulating the quality of water that drains from farmland into the rivers. With NO3 levels increasing in the local rivers, the DMWW anticipates new capital investments of $76-183M for expanded and updated denitrification technology. While the lawsuit was thrown out, it drew enough attention to water quality concerns that the first bill signed into law in the 2018 Iowa legislative session provides $282M over 12 years to farmers and municipalities for water quality improvements.
Changing crops grown, crop management practices and animal agriculture operations can decrease N and pathogen export from farms. Cover crops, perennial living mulch, and integration of forage grassland can reduce drainage and NO3–N leaching [15, 16]. Studies have suggested that changes in land use and vegetative cover affect water uptake patterns that may impact the hydrologic balance on a large scale [17]. However, many of these practices are costly to agricultural producers, and it is not clear who should absorb costs associated with protecting downstream water quality, nor what incentive or policy structures will be most cost-effective [18].
Changing land use to reduce the number of acres in row crops will also adversely affect the net production of both grain and corn-based biofuel. There are other sources of carbon feedstock for ethanol production; corn stover is a readily available feedstock for cellulosic ethanol fermentation. However, soil productivity and water quality could be adversely affected by poor residue management practices: when stover is harvested rather than left on the soil surface, the soil is substantially more vulnerable to erosion. Soil erosion, with streambank erosion, causes excess sediment and nutrient problems, costing billions per year [19]. Also, the amount of harvestable corn stover is not uniform in Iowa [20] when erosion rates are considered as a guideline for sustainable stover harvest. Thus, a complex tug-of-war exists in Iowa between water quality, agricultural production for food, and production of bio-based fuels. The volumes of food and fuel produced, combined with the magnitude of their impacts on downstream water quality, motivate the search for solutions that effectively manage these tradeoffs. The development of models, analysis tools and interdisciplinary approaches for identifying these solutions will have a broader impact on their transferability to similar FEW nexus problems in other geographic contexts.
Supervised by the core participants and other participating faculty, trainees will conduct research on projects with three themes:
Theme 1 – Technologies and practices to increase agriculture’s contributions to energy supply while reducing its negative impacts on water quality and human health;
Theme 2 – Data science to increase crop productivity within the constraints of sustainable intensification;
Theme 3 – Decision sciences to manage tradeoffs and promote best practices among diverse stakeholders.
Financial support from the Traineeship will be targeted to recruit students for core research projects at the FEW nexus. Additional trainees will be supported by related projects funded by other sources. For each topic, initials signify the core participants or other faculty in the multidisciplinary group of researchers.
Core projects
Theme 1
Microbial carbonate precipitation during anaerobic digestion of manure: A strategy for carbon removal and sequestration within a food-water-energy framework (RB)
Livestock production is a source of water pollution and greenhouse gas emissions (GHG) [21]. Open lagoons play a large role in mitigating water pollution but do not address methane emissions, exacerbating the problem of GHG emissions. Covering lagoons mitigates emissions and the resulting methane (CH4)- rich biogas is a renewable energy source [22]. However, the CH4 is diluted with CO2, detracting from its use in power generation and reducing its GHG benefits. If CO2 in biogas could be removed and sequestered, a system that simultaneously promotes sustainable food production, clean water, and renewable energy generation can be envisioned.
We propose to couple anaerobic digestion (AD) and microbial carbonate precipitation (MCP) to transform conventional livestock production into a system of carbon dioxide removal and reliable sequestration (CDRRS) [23] with additional benefits in water quality and renewable energy (see Fig. 4). AD is an effective method for breaking down organic material into CH4 and CO2 [24, pp. 245-249]. MCP is the byproduct of many common microbial metabolic activities [25], including AD[26]. Atmospheric CO2 is via photosynthesis to produce crops, such as corn. The grain feeds cattle and the resulting manure is digested to CH4 and CO2, the latter reacting with silicate minerals via MCP to produce calcium carbonate (CaCO3) and silicon oxide (SiO2), solids that are recycled to the land. The concept has been demonstrated with wollastonite minerals [27]. In the proposed study, biochar from the pyrolysis of biomass serves as provides silicate to the process. Spent biochar along with carbonate and manure digestate becomes soil amendment. The integration of AD and pyrolysis enhances CDRRS by sequestering carbon as both carbonate and solid carbon. Biochar addition to ADs alleviates ammonia inhibition and improves CH4 yields [28].
The student on this project will: (1) collect, analyze, and organize data relevant to the proposed CDRRS; and (2) perform economic and environmental analyses of the system through process modeling. Some of these data will be landscape scale, such as geographical distribution of livestock operations and the amount of manure generated. Other data will be process-specific such as reaction rates and product yields from AD, MCP, and pyrolysis. Much of the data is already available in the scientific literature. How- ever, experiments are needed to generate data on release of CO2 during AD and its sequestration via MCP. AspenPlus [29] will be used to develop a process model for the proposed system. Simapro [30] will be used for life cycle modeling. Decision theory [31, 32] will be used to calculate expected utility of the proposed CDRRS and other approaches to managing manure to avoid water pollution and GHG emissions.
Theme 2
Cyber-physical transformation of poultry egg production (SR, SS)
Poultry eggs are one of the world’s most widely consumed, nutrition-rich, cost-effective, and high-demand animal protein products [33] with one of the smallest environmental footprints based on high feed conversion efficiency. Their production requires energy embodied in the feed and to manage the henhouse environment for bird and worker health while affecting water quality via manure and the wastewater from washing. The current nationwide transition from caged to cage-free operations, in response to consumer concerns about bird welfare, creates challenges to sustainability and resilience. Heat (in summer), moisture (in winter), harmful gases, and dust are crucial operational issues for maintaining a quality indoor environment for birds and workers. Within the barn, hens generate eggs, feces, heat, water vapor, and CO2 based on their metabolic and thermoregulatory processes. Decomposition of fecal waste generates further heat plus noxious gases such as ammonia (NH3), which can be detrimental to bird and human health. Bird activities will also generate particulate matter (PM) which can negatively affect the health of the occupants. This is particularly the case with cage-free housing where bird dust bathing, wing flapping, and foraging on the floor create additional bacteria-laden PM emissions. While aviary birds have the freedom to move and exercise their natural behaviors, they also experience higher mortality rates [34] and less efficient feed conversion, resulting in poorer utilization of energy and water.
Decisions concerning production system design and operation at multiple temporal and spatial scales affect the energy and water used to produce feed and maintain the hen habitat, and have an impact on water quality. Top-level decisions on a decadal time scale are needed for barn design, housing system choice, and material management systems. Mid-level decisions (i.e., months to years) inform hen breed selection and flock plus waste management practices. Lower-level decisions, on the day-to-second scale, include adjustments to feed formulation, operation of the heating and ventilation systems, and any actions necessary to maintain feed and water delivery, waste removal, egg collection, and hen health.
Significant opportunities exist for transforming and improving cyber-based decision processes within egg operations. This project will employ next-generation cyber-infrastructure to collect and decipher heterogeneous data streams associated with barn environment, bird health and behavior, maintenance and animal welfare activities, and food productivity in a spatiotemporally distributed manner. Decision support will be accomplished through vastly increased data collection, modeling, analysis, and visualization to understand the poultry-energy-water system behaviors. The novel henhouse environmental control system will feature explicit modeling of the thermodynamics, moisture, airflow and material conversion as enabled by the sensing and data analytics. Sampling schemes at different temporal and spatial scales will be developed to provide inputs to the algorithms. Upon initial data curation, feature extraction algorithms will be employed to perform dimension reduction and pattern discovery from high-frequency time-series data using a recently reported nonlinear feature extraction tool, called Symbolic Dynamic Filtering [35]. Visual displays of the outputs will be generated for various types of decision support [36]. A data hub for inter-farm comparisons will allow multi-dimensional performance metrics to be integrated along multiple temporal and spatial scales [37] for more robust operation along the bird flock lifecycle.
Theme 3
Improving perennial energy crop representation in agroecosystem models to inform crop and energy production (EH, AK, MS).
Iowa is home to the largest commercial plantations of miscanthus for energy in the US. A current USDA- NIFA funded project will determine the extent to which miscanthus improves nutrient cycling and ecosystem services compared to annual crops grown in farmed potholes. Potholes are agroecological “hot spots” characterized by low-lying, poorly drained, sub-field regions that are disproportionately vulnerable to poor crop production and impaired ecosystem services [38]. Annual crops typically planted in potholes are highly susceptible to excess moisture stress, which leads to poor yields, financial loss, and adverse environmental impacts [39, 40]. As climate patterns become increasingly variable, farmed potholes will become even more exposed to risk [41]. This high-risk/high-reward project will monitor farmed potholes managed with either a corn/soybean rotation or miscanthus. Crop production, water depth, and quality, greenhouse gas emission and profitability of the cropping systems will be assessed by collecting discrete and continuous field data, then integrating them into agroecosystem and profitability models [40, 42] to identify parts of fields where both profitability and environmental performance can be improved by perennials.
A related collaborative project with the University of Iowa uses on-farm data from commercial miscanthus plantations, coupled with novel experimental data from the world’s first replicated chronosequence experiment on miscanthus, to improve perennial grass representation in the agroecosystem models Agro- IBIS [43] and APSIM [44]. Improved model simulations will support decisions on crop management in the UI commercial plantations, expected fuel quality (e.g. nitrogen content) and quantity (biomass productivity), expected profitability based on quality and quantity of biomass, and environmental performance of miscanthus fields compared to corn and soybean production.
The student on this project will collect data on biomass production, biomass quality, profitability and water quality from the commercial plantations as well as commercially farmed prairie potholes described in a related project below. By combining agroecosystem, biogeochemical, financial and logistical modeling with outreach to land managers, better decisions on land and crop management, fuel procurement and infrastructure development are envisioned.